Keywords:
By Remi Magnan
Low drug efficacy and safety concerns are the main reasons for late-stage withdrawal of drugs in clinical trials and account for 87% of all phase III submission failures. [1] Toxicity towards certain organs like the heart, liver or kidneys plays a central role in many of these unsuccessful trials. For the monitoring of tissue-specific toxicity, human induced pluripotent stem cells (hiPSC) are increasingly used as a powerful tool to develop cell models, since they are more relevant, scalable and reproducible model systems compared to traditional animal models and standard immortalized cell lines. Production, handling and differentiation of iPSC and other stem cell-derived models is very time-consuming and greatly benefits from automation. This article explores some of the factors to consider when automating for stem cell handling and differentiation.
Human stem cell models are increasingly being used in drug development and toxicity testing.
What limits our output when it comes to stem cell differentiation and maintenance?
Stem cell protocols that involve manual steps may work fine on a small scale, but can be difficult to control and reproduce at large scales. Stem cells are particularly demanding ‘creatures’ that require constant attention and careful handling. Their growth rates and differentiation stages can be unpredictable, often occurring at inconvenient times such as weekends, late at night, or during holidays. The repetitive and tedious nature of manual tasks such as plate coating, seeding and media exchange creates many opportunities for human error, even with highly skilled staff. Staff availability for these tedious and often inconvenient manual tasks can become a significant issue when scaling up for a screen or large study. During particularly lengthy processes such as stem cell differentiation (which can take weeks or even months to complete), any errors or variability introduced along the way can result in costly setbacks and delays.
Development and optimization of robust stem cell differentiation protocols can be a lengthy and expensive undertaking. The extensive work it takes to guide differentiation into target tissues severely limits the number of experiments that can be carried out at the same time. The level of experience needed to obtain successful and consistent results is an important concern, especially when expanding operations and taking on new team members. Training costs can be very high – in terms of time, money, or both. When optimizing protocols for large-scale studies, screens or production runs, consistent training, error-proofed protocols, and increased capacity to process more cells are crucial ingredients for success.
Standardization of stem cell protocols within the lab and across different labs and operators is essential for reproducibility and reliability. Even when everyone is ostensibly following the same protocol, great variability can be observed in the way different operators perform certain tasks such as making up buffers, coating plates, seeding to replicate wells, etc. Such variability may be acceptable during research and early development phases, but lack of standardization can become extremely problematic when it comes to quality management, especially when operations are scaled up and handed over to a screening or manufacturing group.
How your lab can benefit from automated stem cell handling
Many of the described problems can be diminished or even completely eliminated by automation.
One big upside of robotics is the capacity to handle many cells at the same time, which can drastically improve a lab’s ability to generate more (and more reliable) data without having to hire additional staff. Unlike humans, who enjoy regular sleep, free weekends, and the occasional holiday, robots can work continuously without a break and will do the work the same way, every time.
Studies have shown that robotics can significantly improve protocol reproducibility. For example, Joannides et al. explored the capability of automated mechanical passaging (AMP) to expand human embryonic stem cells (ESC). [2] Their analysis revealed that their cells were more uniform in colony shape and colony numbers than the manual control.
On the level of individual protocols, automation is able to help with many aspects of stem cells work, including plate coating plating, seeding, and media exchanges (Figure 1).
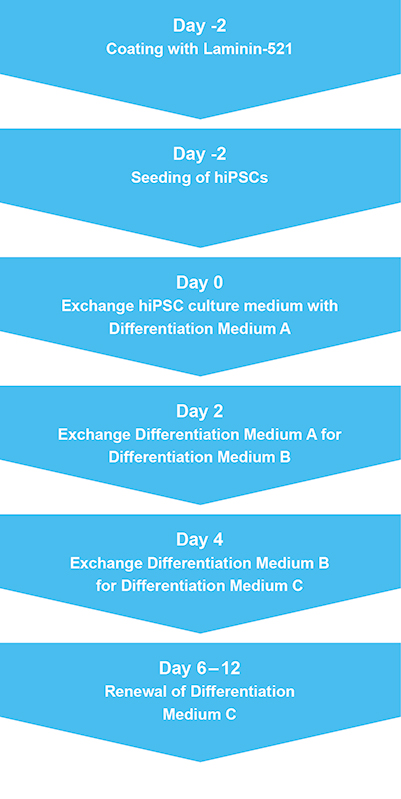
Figure 1: A typical stem cell differentiation workflow
Successful applications of automated stem cell cultures
As automation technology has improved, the use and reliability of automated platforms for stem cell applications has increased steadily over the past decade or so. In an early report, Thomas et al. successfully shifted the manufacturing of a human neural stem cell line used for stroke therapy. [3] The parameters of the resulting cells (viability, growth rate, immunoreactivity and their transcriptome) were all within the strict specifications of the cGMP guidelines for stem cells.
A few years later, Kato et al. evaluated the possibility of expanding primary cell lines using a bench top platform with a footprint not exceeding 0.5 square meters. [4] These cells were successfully differentiated into fibroblasts and bone marrow stem cells, which were able of ectopic bone formation. The compact size of the platform made it practical to host in smaller labs and facilities.
In 2015, a workstation was successfully used to cultivate and passage suspension cultures from four different cell lines, demonstrating the feasibility of this platform for automation of floating cell culture. [5] The authors concluded that their system was fully capable of replacing manual methods.
Requirements and features of cell culture automation
Which components does an automated stem cell workstation need? There are several critical elements for successful automation of stem cell processes, as well as a number of useful accessories that can be integrated to expand capabilities even further.
The basic features include:
- Robotic arms. These are essential both for moving various culture dishes and containers around the workstation, and for transferring liquids and cell suspensions from one receptacle to another. In the case of liquid handling, the arms are outfitted with pipettes. The number of individual pipette tips that the robotic arm can accommodate determines how many wells of a plate can be serviced at the same time.
- Safety cabinet with ample work deck. The safety cabinet is the space where the action takes place and the main operations are performed. For stem cell growth and differentiation, a sterile environment provided by a high-quality safety cabinet is obligatory.
- Worktable. The worktable provides the surface for all processes. It needs to be large enough to store materials (plates, reagents, tip boxes, etc.) while also being configurable to support a maximum number of operations and protocols.
- Incubator. Most of the time, the stem cells are kept in the incubator, which ensures the appropriate environment for their survival. For optimal stem cell growth and maintenance, a humidified atmosphere with O2 and CO2 gas control is essential, as is precise temperature regulation. An “open” liquid handling platform is usually needed to enable integration with a standard tissue culture incubator and other important peripheral equipment.
Besides these fundamental features, there are other accessories that can be built into automated stem cell workflows to add more utility and increase the overall value of the system. Examples for such additions are microplate readers as well as cytometers (for automated measurement of cell confluence), cell separators, shakers and carousels for disposable pipette tips. Depending on the demands, one can add different modules to create an individualized system with strengths matching the requirements.
Conclusion and outlook
Automating stem cell protocols for medium-to-high throughput applications like toxicity screening can significantly increase cost-efficiency and improve process control. The trend is continuing towards minimizing manual intervention and ideally implementing complete workflow automation when possible. Automation can save you time, free up valuable staff, and improve the reliability and reproducibility of results. Overall, this will lead to more efficient use of materials and human resources, and also enables quicker development of new protocols and applications.
Do you want to learn more? Our application note “Differentiation of human induced pluripotent stem cells into cardiomyocytes” describes the adaptation of the Pluricyte Cardiomyocyte Differentiation Kit protocol for 96-well plates using the Fluent Automation Workstation to create a screening platform for compound-related cardiotoxic effects.
References
[1]J. Arrowsmith, Phase III and submission failures: 2007–2010,Nat. Rev. Drug Discov. 2011, 10, 87
[2] A. Joannides, C. Fiore-Hériché, K. Westmore, M. Caldwell, A. Compston, N. Allen, S. Chandran, Automated Mechanical Passaging: A Novel and Efficient Method for Human Embryonic Stem Cell Expansion, Stem Cells 2006, 24 (2),230-235
[3] R. J. Thomas, A. D. Hope, P. Hourd, M. Baradez, E. A. Miljan, J. D. Sinden & D. J. Williams; Automated, serum-free production of CTX0E03: a therapeutic clinical grade human neural stem cell line. Biotechnol Lett. 2009, 31(8), 1167-72
[4] R. Kato, D. Iejima, H. Agata, I. Asahina, K. Okada, M. Ueda, H. Honda, H. Kagami; A compact, automated cell culture system for clinical scale cell expansion from primary tissues Tissue Eng Part C Methods. 2010, 5, 947-56
[5] R. Lehmann, J. C. Severitt, T. Roddelkopf, S. Junginger, K. Thurow; Biomek Cell Workstation: A Variable System for Automated Cell Cultivation, J. Lab. Autom. 2016, 21(3), 439-450
About the author
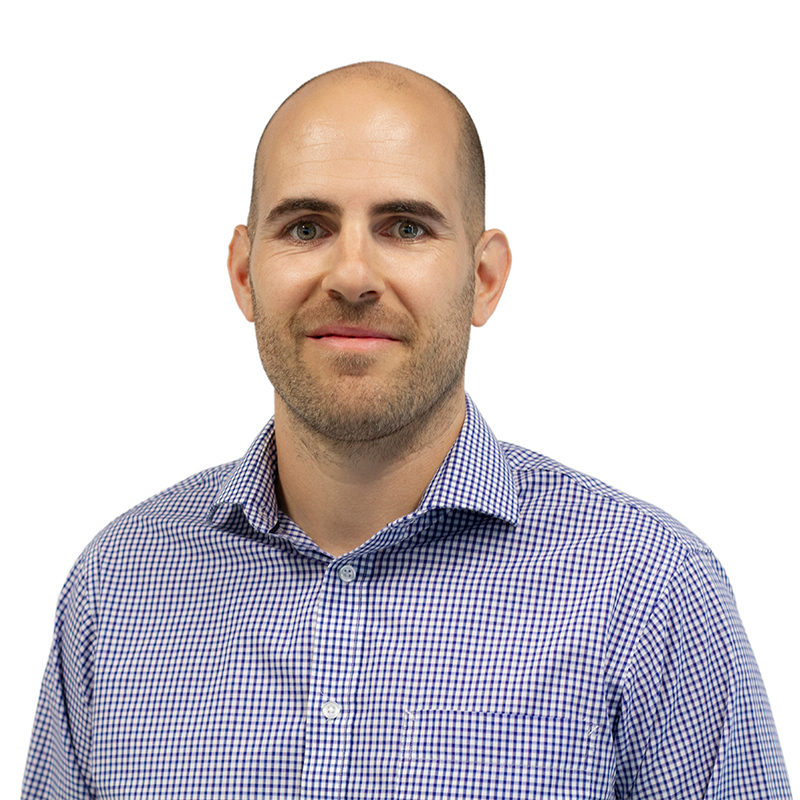
Remi Magnan
Remi is Associate Director Cellomics & Proteomics, leading a group supporting automation of a broad range of applications areas including cell biology, drug discovery, synthetic biology and bioprocessing. Prior to joining Tecan, he worked as a senior scientist and team leader in drug discovery and drug development organizations both in the United States and Europe. Remi is a pharmacologist by training, holding a Ph.D. from the University of Toulouse, France.