Keywords:
By David Wold
Spatial biology, or spatial omics, is the ambitious term covering the science and technologies that allow researchers and clinicians to collect, explore, and analyze spatially resolved information on the interactions of individual molecules, cells, tissues, organs, and organisms, in three dimensions, over time.1-3 An introduction to spatial omics and its manifold challenges can be found in our first article of this series. Let us now look at some of the specific challenges associated with the standardization of the spatial omics workflows, taking spatial transcriptomics as an example.4
Fluorescent Imaging - Immunofluorescence of cancer cells growing in 2D with nuclei in blue, cytoplasm in red and DNA damage foci in green.
Standardizing spatial omics technologies: Nurturing the ecosystem
With the manifold combinations of different sample types, hybridization methods, and image collection and analysis options available, any vision for global standardization of spatial omics might seem practically impossible. However, the commercialization of academic laboratory methods such as MERFISH and RNA seqFISH has significantly accelerated the adoption of spatial omics technologies generally.5-10
In effect, over the past decade, numerous companies have introduced automated platforms, standardized protocols, and integrated analysis tools that have made these complex methods more accessible to researchers. These include Vizgen, Akoya, Nanostring, 10X Genomics, Illumina and others, all of whom have developed comprehensive solutions that combine hardware, reagents, and software.
This commercial development has been continuously refined through academic feedback, creating a dynamic ecosystem of innovation. New startups are continuously emerging with novel solutions spanning reagent kits, automation systems, and AI-powered analysis platforms, expanding the possibilities for spatial analysis.
From an academic and intellectual property perspective, while commercialization offers obvious advantages, such as improved reproducibility and easier access to advanced technologies, the reliance on proprietary systems also risks creating potential barriers. First, expensive proprietary reagents and instruments may restrict access for many researchers. Second, closed systems can impede scientific progress by preventing researchers from modifying or combining methods for novel applications. For instance, undisclosed probe sequences in commercial kits may prevent the development of new multi-omics workflows.
Additionally, proprietary data formats can lock users into vendor-specific software ecosystems, limiting data interoperability and analysis options. In addition, the parallel development of similar methods in academic and commercial settings can create confusion about which protocol version generated particular results, potentially affecting reproducibility.
Therefore, to ensure sustainable growth in spatial omics, a balance must be struck between commercial development and open science. Moving forward, fostering productive academic-industry collaborations will be essential to address key challenges like cost and throughput, ultimately making spatial omics more accessible to the broader scientific community.
Tecan's strategy is fundamental in addressing this need for balance. Its modular approach to developing liquid handling platforms allows it to assemble individual modules, protocols, and scripts as required. This flexibility is beneficial for both innovative basic academic research and more routine, commercially-driven applications.
Spatial transcriptomics: A recap of the challenges
Spatial transcriptomics studies how transcripts from hundreds of different genes are expressed across a biological tissue, with single-cell resolution.4 The ultimate goal of spatial transcriptomics is to measure the abundance of every gene and gene isoform in three dimensions at subcellular resolution in a whole tissue sample.2 However, while bulk and single-cell sequencing can provide a wealth of information about cells at a transcriptomic level, these methods involve breaking down the tissue to access and separate individual cells. As a result, this makes it difficult to comprehensively characterize a cell whilst also understanding its precise location within the tissue. In addition, most current technologies focus on histological sections of various thicknesses, so they are typically 2D rather than 3D methods.
To overcome these limitations, various promising spatial transcriptomics workflows have been developed that are based on the well-established technique of fluorescence in situ hybridization (FISH). For example, combinatorial FISH can be used with single-molecule imaging to quantify the number of mRNA molecules present in a given area of a tissue.2,5,6 This combination of techniques can detect single molecules per cell and can theoretically be used to profile millions of targets instead of tens or hundreds. Molecules are detected through hybridization and identified according to patterns of fluorescence signal. Multiplexed error robust FISH (MERFISH*), sequential FISH (seqFISH), and seqFISH+ use combinatorial labeling schemes to assign each transcript to a barcode, consisting of a subset of hybridization probes that are detected in repeated cycles of imaging and release.2,5,6
The workflow complexity can be addressed with techniques such as MERFISH, which allows spatially resolved gene expression profiling of single cells in intact biological samples by assigning error-robust barcodes to individual RNA species, followed by barcode detection through sequential imaging (see Fig. 1).7-10
Figure 1. Simplified overview of the MERFISH workflow for successive hybridizations (adapted from Chen et al., 2015, and Moffitt et al., 2016).
Image-based approaches to spatial transcriptomics such as that offered by MERFISH offer the ability to quantify not only the copy number of RNAs within cells but also the intracellular RNA location and the spatial organization of cells within cultures or tissues.8-11 Indeed, the advanced MERFISH method first published by Zhuang’s team in 2016 allows gene expression profiling of ∼40,000 human cells in a single 18 h measurement.
The order of magnitude increase in throughput now seen with these techniques allows us to pinpoint transcriptionally distinct cell types within ever-larger tissue areas. Access to such exquisite granularity is crucial to our understanding of tumor heterogeneity, for biomarker discovery and development, and for creating integrated atlases of cell types across organs and whole organisms.
Standardization of liquid handling for spatial transcriptomics
With the increased throughput enabled by emerging spatial transcriptomics technologies, there is a higher potential for error, particularly in the liquid handling aspects of these intricate workflows. The precision of liquid handling directly impacts data quality, reproducibility, and the ability to detect rare transcripts across tissue sections.
At the front end of the workflow, during tissue preparation and fixation, precise reagent volumes ensure uniform tissue preservation and maintain spatial integrity, and the application of permeabilization buffers requires exact timing and volumes to maintain tissue morphology, while allowing efficient access to RNA molecules.11
Further downstream, both imaging and sequence-based transcriptomics techniques rely heavily on the successful and reproducible hybridization of primary probes, followed by multiple rounds of hybridization with secondary probes, as seen in the MERFISH example above (Fig. 1).
The sheer number of rounds of hybridization, each round requiring the completion of multiple steps — hybridization followed by imaging, washing, bleaching and reprobing — means that these complex techniques would not be reliable unless the liquid handling is accurate to within sub-microlitre tolerances from one cycle to the next. Even minor variations in probe concentration or washing stringency can significantly affect signal-to-noise ratios and detection sensitivity.5
Innovations in microfluidic technologies and automated liquid handling systems continue to improve spatial transcriptomics workflows. These platforms offer:
- Precise volume control down to nanoliter scales
- Consistent reagent dispensing across tissue sections
- Reduced operator-dependent variation
- Standardized washing protocols
These advances promise to enhance reproducibility while reducing reagent consumption and experimental costs. In our final article in this series, learn more about how Cavro® liquid handling solutions can be used to implement your own cutting-edge spatial omics platform.
*The MERFISH technique was pioneered in the laboratory of Xiaowei Zhuang, Harvard University, Cambridge, Massachusetts.8,9 The MERFISH patents are owned by The President and Fellows of Harvard College. Patent No. 11,959,075, relating to robust multiplex imaging of RNA and its spatial organization in a sample, is exclusively licensed to Vizgen.12,13
References
1. Alexandrov, T., Saez-Rodriguez, J., & Saka, S. K. (2023). Enablers and challenges of spatial omics, a melting pot of technologies. Molecular systems biology, 19(11), e10571.
Pubmed: https://pubmed.ncbi.nlm.nih.gov/37842805/
DOI: https://doi.org/10.15252/msb.202110571
2. Bressan, D., Battistoni, G., & Hannon, G. J. (2023). The dawn of spatial omics. Science (New York, N.Y.), 381(6657), eabq4964.
Pubmed: https://pubmed.ncbi.nlm.nih.gov/37535749/
DOI: https://doi.org/10.1126/science.abq4964
3. https://pubmed.ncbi.nlm.nih.gov/?term=%22spatial+omics%22&sort=date
(Accessed 21 May 2024)
4. Marx, V. Method of the Year: spatially resolved transcriptomics. Nat Methods 18, 9–14 (2021).
DOI : https://doi.org/10.1038/s41592-020-01033-y
5. Eng, C. L., Lawson, M., Zhu, Q., Dries, R., Koulena, N., Takei, Y., Yun, J., Cronin, C., Karp, C., Yuan, G. C., & Cai, L. (2019). Transcriptome-scale super-resolved imaging in tissues by RNA seqFISH. Nature, 568(7751), 235–239.
Pubmed: https://pubmed.ncbi.nlm.nih.gov/30911168/
DOI: https://doi.org/10.1038/s41586-019-1049-y
6. Lu, T., Ang, C. E., & Zhuang, X. (2022). Spatially resolved epigenomic profiling of single cells in complex tissues. Cell, 185(23), 4448–4464.e17.
Pubmed : https://pubmed.ncbi.nlm.nih.gov/36272405/
DOI : https://doi.org/10.1016/j.cell.2022.09.035
7. Chen, K. H., Boettiger, A. N., Moffitt, J. R., Wang, S., & Zhuang, X. (2015). RNA imaging. Spatially resolved, highly multiplexed RNA profiling in single cells. Science (New York, N.Y.), 348(6233), aaa6090.
Pubmed : https://pubmed.ncbi.nlm.nih.gov/25858977/
DOI : https://doi.org/10.1126/science.aaa6090
8. Moffitt, J. R., Hao, J., Wang, G., Chen, K. H., Babcock, H. P., & Zhuang, X. (2016). High-throughput single-cell gene-expression profiling with multiplexed error-robust fluorescence in situ hybridization. Proceedings of the National Academy of Sciences of the United States of America, 113(39), 11046–11051.
Pubmed: https://pubmed.ncbi.nlm.nih.gov/27625426/
DOI: https://doi.org/10.1073/pnas.1612826113
9. Moffitt, J. R., & Zhuang, X. (2016). RNA Imaging with Multiplexed Error-Robust Fluorescence In Situ Hybridization (MERFISH). Methods in enzymology, 572, 1–49.
PubMed : https://pubmed.ncbi.nlm.nih.gov/27241748/
DOI : https://doi.org/10.1016/bs.mie.2016.03.020
10. Xia, C., Fan, J., Emanuel, G., Hao, J., & Zhuang, X. (2019). Spatial transcriptome profiling by MERFISH reveals subcellular RNA compartmentalization and cell cycle-dependent gene expression. Proceedings of the National Academy of Sciences of the United States of America, 116(39), 19490–19499.
Pubmed: https://pubmed.ncbi.nlm.nih.gov/31501331/
DOI: https://doi.org/10.1073/pnas.1912459116
11. Ståhl, P. L., Salmén, F., et al., (2016). Visualization and analysis of gene expression in tissue sections by spatial transcriptomics. Science (New York, N.Y.), 353(6294), 78–82.
Pubmed: https://pubmed.ncbi.nlm.nih.gov/27365449/
DOI : https://doi.org/10.1126/science.aaf2403
12. https://patents.google.com/patent/US11959075B2/en?oq=US11959075
(Accessed 12 August 2024.)
13. https://vizgen.com/vizgen-issued-a-new-us-patent-for-its-merfish-technology/
(Accessed 12 August)
Keywords:
About the author
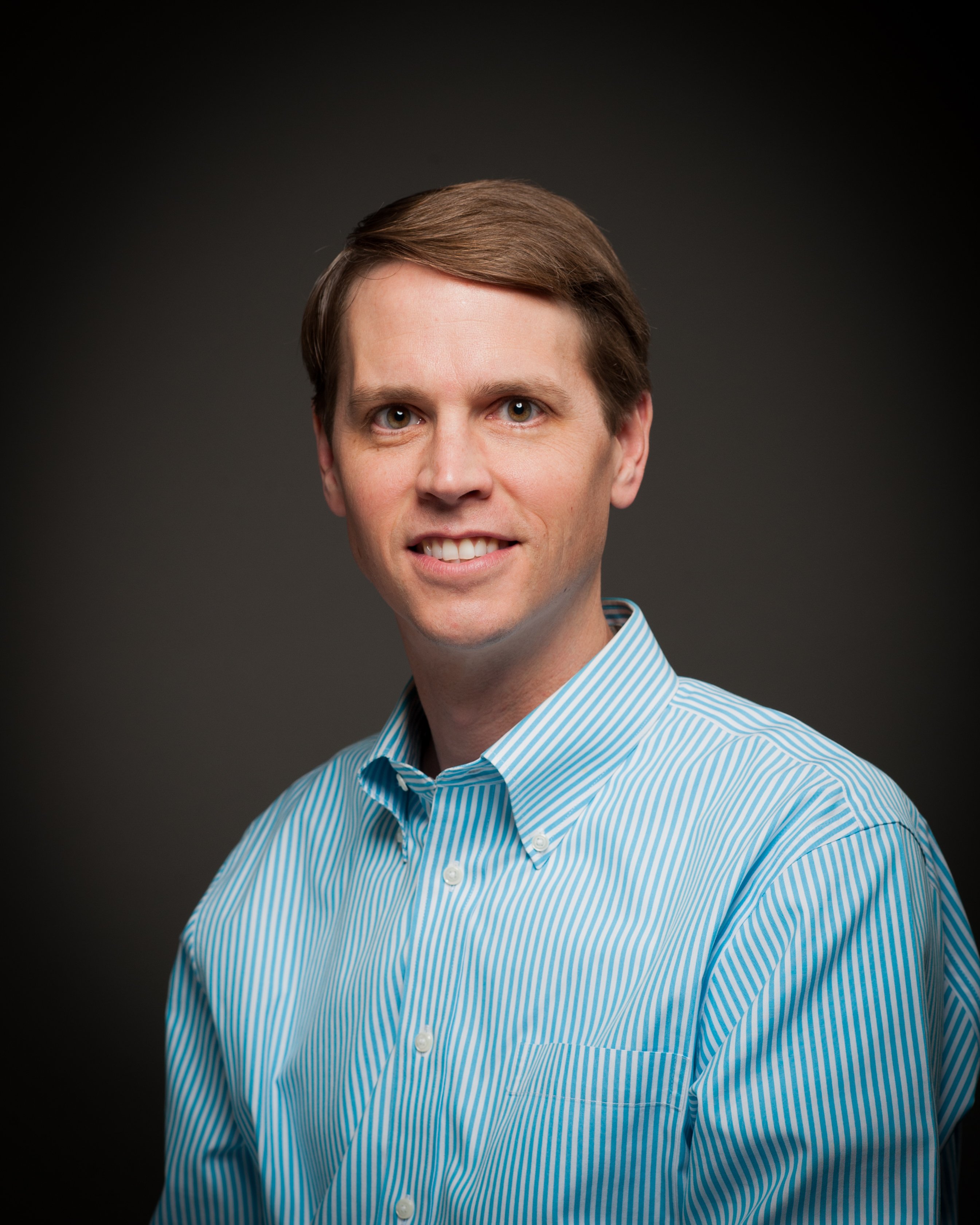
David Wold
David Wold is the Customer Operations Manager for the Partnering Business in the Components Marketing Team. In this role, he oversees a team responsible for the support and satisfaction of Tecan’s top Components customers. Since 2005, David has helped Tecan customers meet their liquid handling challenges across a broad range of technologies and applications. He brings understanding and expertise to help customer choose components to meet their needs, provide maximum reliability and outstanding performance.