Keywords:
By Remi Magnan
Research using stem cells and stem cell-derived models holds huge promise for drug discovery and therapeutic applications. However, creating, characterizing, maintaining and expanding stem cell-derived models and therapeutics can be a time-consuming and error-prone bottleneck. The emergence of genetically engineered induced pluripotent stem cells (iPSC) has opened the door to more relevant and reproducible human model systems and scale-up strategies, yet many challenges remain when it comes to the practical application of iPSC in the lab. In this article we take a look at the advantages that iPSC technology brings as well as some of the main challenges that must be addressed to increase research output and quality.
Stem cells are the key to unlocking the regenerative abilities of the human body.
What are induced pluripotent stem cells used for?
Induced pluripotent stem cells (iPSC) are artificial stem cells with similar characteristics to those of embryonic stem cells (ESC). In contrast to ESC, which must be obtained from the inner cell mass of embryos at the blastocyst stage, iPSC can be created from adult somatic cells — typically obtained from skin or blood samples. Groundbreaking work in the mid-2000’s to identify key transcriptional regulators and pluripotency genes paved the way for the technology to reliably reprogram adult cells into iPSC using genetic engineering. [1,2]
What makes iPSC so promising?
A defining property of stem cells is their pluripotency—the ability to differentiate into virtually any bodily cell type. Compared to ESC, iPSC have the added advantage that they can be readily obtained from human samples without the associated ethical issues.[4] This makes them ideal candidates for the development of human cell models for research and drug screening, as well as for use in regenerative medicine, to repair destroyed tissue or even grow into new functioning organs. In addition, stem cells can be propagated in culture indefinitely and are therefore a renewable source that can be used to generate large quantities of cells reliably and reproducibly. With those characteristics, stem cell-derived products and therapies can be manufactured at the scale and quality needed to enable life changing treatments and novel therapies. Various clinical trials are ongoing to demonstrate the use of iPSC in medicine.[3] However more research is needed to bring this technology into common clinical practice.
The potential for iPSC applications is vast, spanning from basic research to drug discovery to regenerative medicine and the development of novel disease therapies. For example, iPSC are used to study the molecular mechanisms and pathways important for differentiation of tissues during embryonic development. Differentiated iPSC can be useful as models to better understand disease mechanisms or to screen candidate drugs for desired effects on specific cell types and tissues. In addition, iPSC-based therapies are being explored for their potential to treat diseases related to aging like Parkinson’s, macular degeneration and heart disease.[5,6]
Challenges in the practical application of stem cells in the lab
Growth, handling, and controlled differentiation of iPSC and ESC are labor-intensive processes. Differentiation alone can take several weeks (or even months), and typically requires numerous manual media exchanges and other supportive actions like cell seeding and plate coating. [7] This can severely limit the throughput of any assay and the plate format you can work with. Many differentiation protocols are dependent on expensive media, growth factors and other supplements, so each differentiation can be very costly to produce, even if performed on a relatively small scale. Sterility is also critical when working with iPSC and ESC cultures, many of which require antibiotic-free culture media for optimal performance, making them highly susceptible to contamination. Contamination and workflow errors can significantly delay your project and incur a large cost because the entire batch may be lost, in which case the long differentiation process may have to be repeated from the start. These factors make stem cell protocols very challenging to optimize, scale up, and produce cost-efficiently.
On top of that, the protocols for iPSC handling are very monotonous and are generally not that exciting to perform for researchers and lab technicians. The large amount of work associated with iPSC — including unavoidable feeds over weekends and holidays — can be one of the biggest hurdles for your research progress and also creates a psychological barrier for new scientists to join the project.
If you are involved in iPSC research, you probably have already thought about ways to make your workflows more efficient. If not, it definitely pays to review the entire process from end to end to identify the most time-consuming and error-prone steps and then explore ways to reduce the amount of intervention needed at those points.
Commercial kits for stem cell differentiation may provide easier workflows and standardized protocols that have been well-optimized, pre-tested, and validated. All of this can save you time, cost, and frustration when getting up and running with a new cell line or differentiation process. Stem cell differentiation kits often contain premixed buffers and difficult-to-source reagents that facilitate reliable differentiation of iPSC into specific cell types like cardiomyocytes, oligodendrocytes or pneumocytes. Nevertheless, while they can shorten protocol development time and provide some additional convenience and security, it’s important to be aware that pre-developed kits cannot save you from much of the labor associated with handling and maintaining iPSC cultures, and have the drawback of being more expensive to use compared to traditional methods.
How to drastically increase your research output
Research with iPSC, and especially the tedious process of differentiation, greatly benefits from automation by computer-controlled robotics for cell and liquid handling.[8][9] This frees you and your staff members from performing monotonous and error-prone tasks such as cell seeding, frequent media changes, and regular feeds. Automation brings greater standardization and control to your process and can significantly improve reliability, helping you avoid small deviations in the differentiation protocol that can have big consequences, including significant process variation and even differentiation of iPSC into the wrong cell type.
Automation potential is not limited to growth and differentiation of iPSC, but can also be applied to the analysis of cell phenotype, confluence and quality. Overall, automation massively speeds up your research and is the most important step to efficiently scale your lab’s output. With just one robot, you can generate the same amount of differentiated iPSC as with a whole team of technicians. This gives you and your group more time to spend on other high-value tasks, such as those that can only be performed manually or require greater intellectual input, e.g. improving experimental design, interpreting experimental results or preparing publications.
To get more information about what stem cell automation can do for your lab, download our application note “Automated maintenance and differentiation of human iPSCs”.
References
[1] K. Takahashi, S. Yamanaka, Induction of Pluripotent Stem Cells from Mouse Embryonic and Adult Fibroblast Cultures by Defined Factors, Cell. 2006 126 (4), 663-676
[2]Stadtfeld M and Hochedlinger K, Induced pluripotency: history, mechanisms, and applications, Genes Dev. 2010 24(20):2239-2263.
[3] J. Braganca, J.A. Lopes, L. Medes-Silva, J.M.A. Santos, Induced pluripotent stem cells, a giant leap for mankind therapeutic applications, World J. Stem Cells 2019 11(7), 421-43
[4] S. Konagaya, T. Ando, T. Yamauchi, H. Suemori and H. Iwata, Long-term maintenance of human induced pluripotent stem cells by automated cell culture system, Scientific Reports, 2015, 5, 16647
[5] Fariss RN, Stambolian D, Abecasis GR, Curcio CA, Swaroop A. Age-related maculardegeneration: genetics and biology coming together. Annu Rev Genomics Hum Genet 2014; 15, 151-171
[6] D. Cyranoski, Reprogrammed' stem cells approved to mend human hearts for the first time. Nature 2018, 557, 619-620
[7] M.K. Conway, M.J. Gerger, E.E. Balay, R. O'Connell, S. Hanson, N. J. Daily,T. Wakatsuki Scalable 96-well Plate Based iPSC Culture and Production Using a Robotic Liquid Handling System, J. Vis. Exp. 2015, 99, e52755
[8] Crombie et al., Development of a Modular Automated System for Maintenance and Differentiation of Adherent Human Pluripotent Stem Cells, SLAS Discov. 2017, 22 (8), 1016-1025
[9] Daniszewski et at. Automated Cell Culture Systems and Their Applications to Human Pluripotent Stem Cell Studies, SLAS Technol. 2017, 23 (4); 315-325
About the author
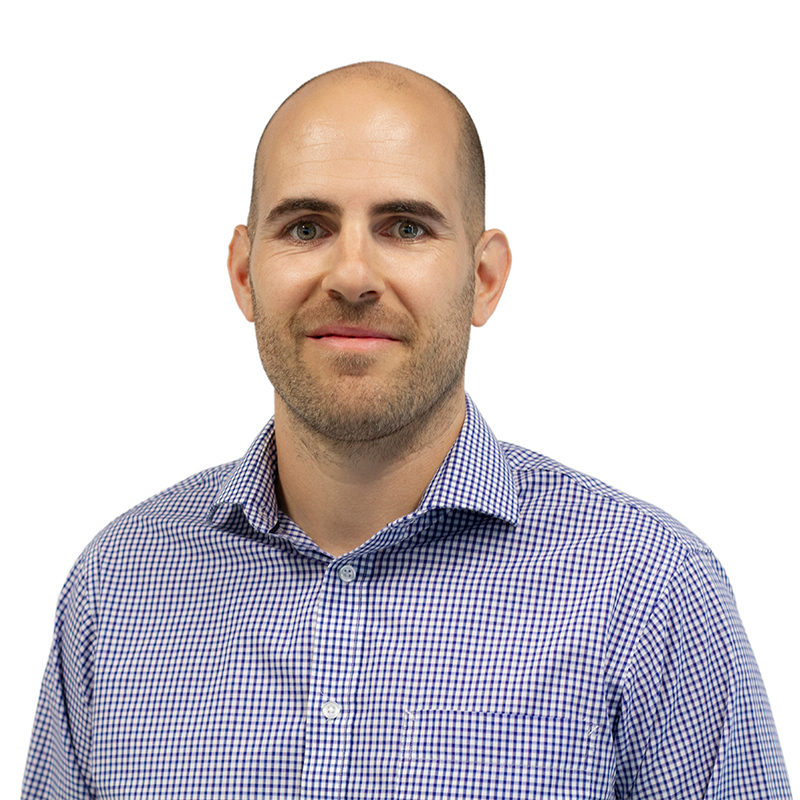
Remi Magnan
Remi is Associate Director Cellomics & Proteomics, leading a group supporting automation of a broad range of applications areas including cell biology, drug discovery, synthetic biology and bioprocessing. Prior to joining Tecan, he worked as a senior scientist and team leader in drug discovery and drug development organizations both in the United States and Europe. Remi is a pharmacologist by training, holding a Ph.D. from the University of Toulouse, France.