By Christian Oberdanner
Live cell imaging is one of the most important techniques in the life sciences today. But behind every great imaging assay, pity the poor scientist grappling with the demands of biological variability and complex kinetic cell assays. Live cell experiments are often synonymous with unsociable working hours, tedious protocols and unrepeatable results. In this blog we explore what it takes to tame automated cell imaging assays and take back control of kinetic experiments to get reliable results more quickly, with fewer errors, and less aggravation.
Real-time cytometry is empowering life science exploration
The popularity of imaging living cells continues to grow year on year. In the last 5 years alone, there have been nearly 8000 scientific publications on live cell imaging—more than in the preceding 40 years combined. (Source: PubMed Database). Why? Because the old adage "seeing is believing" is actually true: images convincingly capture aspects of living cells’ behavior and function that are otherwise difficult or impossible to detect.
The number of publications on live cell imaging continues to rise each year. (source)
Today's cell imaging platforms enable real-time capture of individual cellular events in non-destructive multi-well formats that support higher throughputs and robust, cell-by-cell, statistical analyses.
When image-based cytometry is combined with the capabilities of a multi-mode plate reader, even more insights are possible. With multiple detection modalities, including both top- and bottom-read configurations, you can multiplex more types of assays and make use of novel fluorescent probes to quantify complex dynamic events.
Why live cell experiments get out of hand
Real-time live-cell assays often involve sensitive cell types and complex protocols that can easily go awry—wasting valuable resources and possibly giving you misleading results.
Here are 6 common pitfalls that can send your live cell experiments spinning out of control:
1. Starting with unhappy cells.
Many live cell assays are doomed to fail before they even start because the cells are not in the exponential growth phase or have been subjected to stress prior to the experiment—for example, when transferring from the tissue culture incubator to a slightly different environment in the imager or plate reader.
Check out our previous blogs for more information about the influence of confluence and environmental conditions on live cell imaging experiments, and what you can do to keep your cells happy when preparing for your assay.
2. Experimental complexity.
Kinetic live cell assays can get very complex, very fast. That’s because you need to factor in many different fluorescent probes, time points, dose concentrations, replicates, control wells, cell types, and so on.
The more elaborate the experiment, the more chances there are for variability to creep in and mistakes to be made. To make matters worse, manual steps and complicated instrument control software can significantly increase the likelihood of human error.
Unfortunately, some experimental complexity is often unavoidable—you really do need all those concentrations, replicates and time points! Fortunately, cell imaging platforms are advancing to help us accommodate the necessary complexity, rather than avoid it. Read on to find out how.
3. Failing to adapt to biological variability.
Living cells are inherently variable, which means that to obtain consistent assay results from day-to-day or lab-to-lab, you may need to adjust experimental protocols and timings on the fly. For example, rather than always starting your assay a fixed number of hours after seeding, you may get more consistent results if you wait a variable time period until the cells reach their optimal confluence—say, 80%.
But what do you do if the cells reach 80% confluence when you aren’t in the lab? Quite often, we compromise and start the assay at the wrong confluence rather than go back to the drawing board and re-work the timings.
When you compromise protocol timings to fit your schedule, the assay may still work, but reviewers may question whether your results are biologically relevant.
In the example below, we see how variable factors like percentage cell viability and fluorescent signal may dictate the most appropriate times to add various stimuli or probes, or when to start and stop imaging to ensure you capture the full response.
4. Disturbances and disruptions.
Mechanical or environmental disturbances can trigger cellular stress responses that will alter cell behaviors and responses to stimuli, and may even activate cell death pathways. Common culprits include lid-lifting, harsh addition of reagents or compounds, wash steps, and transfer of plates from one device to another.
5. Scale-up and miniaturization.
Kinetic assays may work fine at the prototype stage, only to fall prey to mistakes when scale-up suddenly amplifies the number of replicates, pipetting steps, samples and compounds.
In addition, scale-up often entails miniaturization, which means dealing with very small liquid volumes. In such cases, evaporation—and by extension, assay duration—becomes a significant concern. At the same time, liquid handling accuracy and precision become even more critical, and the chances of mistakes and cross-contamination are further magnified.
6. Image management challenges.
Depending on the assay complexity, a live cell imaging experiment may generate thousands—or even hundreds of thousands—of images per run. Data storage and archiving can be expensive, and servers quickly become filled to capacity.
If storage space runs out in the middle of a critical run, you risk losing the cells and the entire data set—a big toll in terms of the cost, time and labor you put into your experiment.
For all these reasons, limiting the number of images you need to acquire can be a smart move.
When a simple live-dead assay isn’t so simple
Live-cell imaging assays require a high degree of responsive control. By that I mean that the person running the experiment typically needs to monitor conditions frequently and take action based on cell appearance or some other biological cue, such as intensity of a fluorescent indicator.
To illustrate the challenges of live cell imaging assays and the level of experimental control needed to avoid the pitfalls discussed above, let’s take a look at a relatively simple “live-dead” cell viability assay.
The goal of this assay is to monitor changes in percentage cell viability in response to varying doses of test compound, generating a dose-response curve and IC50 (half maximal viability) for each. To assess viability, we are using a conventional combination of two fluorescent dyes: calcein AM to identify live (metabolically active) cells, and propidium iodide (PI) to detect dead cells (loss of plasma membrane integrity).
Seems pretty straightforward, right? But take another look at the assay schematic. There are several key points in the protocol when biological conditions trigger a particular action.
If you are running the assay without full automation, you will need to assess the cells periodically on a manual microscope to monitor confluence and decide when to add compound. The timings for compound addition, dye addition and PI fluorescence recording are all dictated by the confluence of the cells. Likewise, the ideal imaging start- and stop-times depend on when the cells start to be affected by compound, and when the percentage cell viability bottoms out. Orchestrating all these actions manually or across multiple detection platforms without making any mistakes is not easy.
Maybe this “simple” live-dead assay is not so simple after all!
How to take back control of your live cell experiments
Let’s take a look at some improvements we can make to gain more control and eliminate error.
Improvement 1: Get on-board environmental control.
At the start of the experiment, cells are seeded into a 384-well plate, and left to incubate until they have recovered and reached 80% confluence. Crucially, you need to avoid shocking the cells when transferring them from the tissue culture incubator to the liquid handling station or detection system. This means providing the cells with a stable, on-board, humidified environment (typically 37oC, 5%CO2), where they can equilibrate for a sufficient amount of time before compound is added and measurements are taken.
Improvement 2: Automatically trigger actions using thresholds.
Depending on the health of the cells at the time of seeding, the length of time needed to reach 80% confluence can vary unpredictably. To avoid the hassle of having to adapt your protocol and working hours to suit the cells, the smart solution is to automate confluence determination, and set up a confluence threshold that will automatically trigger subsequent steps.
An automated multi-mode reader and cell imaging platform with this sort of conditional real-time experimental control can minimize the amount of manual intervention needed, while eliminating subjective assessments — triggering additions and reads at the right times, based on accurate quantitative measurements.
Improvement 3: Reduce the number of images acquired.
As shown in the schematic, the assay is configured to run for approximately 48 hours from start to finish. If we run the assay in just one 384-well plate, collecting a single whole-well image in both red and green channels every hour from the point that the dyes have been equilibrated (i.e., hours 17 through 48), we’ll need to collect a jaw-dropping 24,576 images.
Can we do better?
Of course. It turns out that we are collecting many images that are not needed, namely those at the very top and bottom of the dose-response curves. The key to reducing the image number is to use threshold-based conditional programming to collect only the images that are actually needed to generate a reliable curve.
Here’s how it works:
After the cells have been equilibrated with dye mix, we start continuously recording PI intensity using a bottom read (no images required!). We can then automatically detect an increase in fluorescence when dead cells first start appearing, and use an intensity threshold to trigger the 2-color imaging to start from that point onward.
Similarly, if we can calculate percent viability by analyzing the image data in real-time, we can set second threshold which will trigger the imaging to stop when viability bottoms out, in this case at 10%. With both thresholds applied, imaging is carried only from hours 20 through 44.
The result
Automated real-time experimental control reduces the number of acquired images by 5376 — over 20%! That’s a major savings in terms of both experiment time and storage space.
Improvement 4: Create a “walk-away” process.
A final consideration is the amount of hands-on time needed to run the assay from start to finish. As mentioned earlier, the more manual steps you can eliminate the better.
Automating as much of the assay protocol as possible will significantly improve accuracy, decrease variability, reduce the chances of error, and free valuable staff up for more important, less tedious tasks.
The answer? A multi-mode imager with real-time experimental control
The best way to achieve hands-free, error-proof kinetic cell assays is with a multi-mode reader that includes on-board environmental control and lets you perform image-based confluence assessment, intensity measurements, fluorescence imaging and real-time image analysis — all on the same system. And crucially, you need a system that allows you to set up threshold-based conditional responses easily and with no need for programming skills.
Want to learn more?
Find out more about the Spark Cyto live cell imaging microplate reader with real-time cytometry.
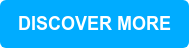
About the author
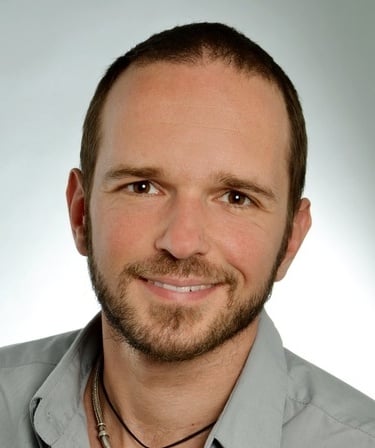
Christian Oberdanner
Dr. Christian Oberdanner is Product Manager at Tecan Austria. He studied molecular biology at the University of Salzburg with a strong focus on cell- and tumor biology during his PhD study. Christian started to work for Tecan Austria as external scientific consultant in 2005 and permanently joined the company in 2006. Since then he held the role of an Application Specialist in the research and development department as well as in the sales and marketing department and since 2015 of a Product Manager. Christian’s priority within Tecan is the multimode microplate reader portfolio.