By Kevin Moore
Like gravity, some phenomena are so integral to our existence that we’re barely conscious of them. Maybe that’s why the research community was largely taken by surprise when it was announced that this year’s Nobel Prize in Physiology or Medicine was awarded to three American scientists for their seminal work on circadian clocks ¹. But consider the synergies with next gen sequencing (NGS) and gene editing technologies, and it becomes clear that the implications of their work are far-reaching.
The Nobel Prize recognizes advances in chronobiology that could give us god-like control over our circadian clocks.
While Nobel prediction pundits pointed to recent high-profile, and no doubt equally Nobel-worthy discoveries (such as CRISPR-based gene editing, the role of PI3K signaling pathways in cancer, and advances in immunotherapy), it seems many of us had simply taken for granted the pioneering discoveries of this year’s laureates: Jeffrey C. Hall, Michael Rosbash and Michael W. Young.
Yet the light shed by Hall, Rosbash and Young on molecular mechanisms of circadian clocks set the stage for significant advances in medicine and the fascinating field of chronobiology, with diverse practical applications in pathology, disease prevention, molecular diagnostics, toxicology, pharmacology and more.
Overlooked, but not over-rated
Virtually all multicellular organisms have evolved ways to synchronize their physiology and behaviors with the Earth’s revolutions. These daily biological oscillations were dubbed “circadian rhythms” by the founder of chronobiology, Franz Halberg, who derived the term from the Latin “circa” (around) and “diem” (day). Circadian clocks are so important that their key mechanistic components have been conserved throughout evolution, from invertebrates to humans. But why should that be?
Circadian clocks enable us to anticipate and prepare for the challenges of our fluctuating environment. They help us wake earlier than potential predators; remain alert and energetic during the day when we need to hunt and forage for food; and maintain a regular sleep cycle, during which we repair and renew the body’s machinery.
Ever suffer from jetlag? Then you have experienced first-hand the unpleasant effects arising from a body clock that is temporarily out of sync with its environment. The consequences can be even more serious when clock misalignments and malfunctions become chronic.
Not one clock, but many
Although we each seem to dance to the beat of a single internal clock, the various tissues and organs of the body keep time to separate peripheral clocks that maintain rhythmic periodicity even in the absence of solar cues. In fact, nearly every cell in your body has its own self-perpetuating clock mechanism, running on a roughly 24-hour cycle. The activity of these clocks is exquisitely coordinated across the body by a master regulator in the brain called the suprachiasmatic nucleus (SCN).
Responding to information from photosensitive cells in the retina, the SCN is able to synchronize its neuronal activity daily with the solar cycle. In turn, information about the length of day and night gathered by the SCN is cascaded to the pineal gland and then to other tissues throughout the body via neuronal and hormonal mediators.
Aside from making us feel unwell, chronic asynchrony of our internal clocks – with each other, with the external environment, or both – can have serious long-term consequences on our health and well being, including the development of behavioral and metabolic disorders, cancer, diabetes and heart disease. This is largely because circadian clocks regulate critical metabolic and endocrine rhythms throughout the body.
“I must govern the clock, not be governed by it.”
— Golda Meir
Understanding what makes us tick
One of the first insights into the genetic components of circadian clocks came in 1971, when Seymour Benzer and his student Ronald Konopka at California Institute of Technology showed that chemical mutagenesis of a single functional gene on the X-chromosome could dramatically disrupt, lengthen or shorten the circadian period of the fruit fly ². Their screen identified 3 alleles of this clock gene, which they aptly named period (per).
Armed with emerging new techniques in molecular biology, this year’s laureates unlocked fundamental mysteries of how circadian clocks keep time at the molecular level. In 1984, Young (at Rockefeller University) and Hall and Rosbash (collaborating at Brandeis University) isolated the D. melanogaster period gene and showed that it could restore circadian rhythms when transformed into arrhythmic fruit flies ³,4.
Demonstrating that per mRNA transcripts and protein (PER) levels oscillated over a 24-hour period, Hall and Rosbash showed that PER had the required hallmarks of a circadian pacemaker component 5,6. Importantly, they postulated that the self-perpetuating nature of the clock involved a negative feedback regulatory loop, whereby PER could inhibit its own synthesis 6. In order for this mechanism to work, PER would need to translocate from the cytoplasm to the nucleus, and the timing of regulation would have to be tunable to adapt to the solar clock. Among other crucial contributions, Young and colleagues investigated pacing of the Drosophila clock, identifying the timeless 7,8 and doubletime 9 genes essential for spatiotemporal control of the mechanism. Together, the work of the laureates sparked a new era in circadian biology that continues to flourish and yield valuable insights.
NGS takes the discovery further
Today, we know that the tightly regulated and finely tuned circadian clock mechanism coordinates the rhythmic expression of thousands of genes critical for our health, wellbeing and survival.
Building on the foundations laid by Hall, Rosbash and Young, the application of new technologies like NGS has transformed our understanding of circadian genomics. The study of circadian proteomics has also flourished. We now understand that regulation of circadian clocks involves a complex network of transcriptional and post-transcriptional controls. This information has led to some potentially paradigm-changing insights 10.
Many questions remain, but the answers are already impacting diverse aspects of our lives, from lifestyle choices to therapeutic drug development, and even the engineering of spacecraft environments.
And who knows? With the advance of gene editing technologies, future applications of chronobiology may well extend to bioengineering our food sources and even ourselves to better adapt to abnormal light levels, artificially altered sleep-wake schedules and even extraterrestrial habitats.
Want to stay updated?
Subscribe to the Tecan blog to receive the latest articles and ideas straight to your inbox.
References
- "The 2017 Nobel Prize in Physiology or Medicine - Press Release". org. Nobel Media AB 2014. Web. 21 Oct 2017, viewed 20 October 2017.
- Konopka RJ and Benzer S. Clock mutants of Drosophila melanogaster. Proc Natl Acad Sci USA (1971) 68(9):2112-2116.
- Bargiello TA, Jackson RF and Young MW. Restoration of circadian behavioural rhythms by gene transfer in Drosophila. Nature (1984) 312:752-754.
- Zehring WA, Wheeler DA, Reddy P, Konopka RJ, Kyriacou CP, Rosbash M and Hall JC. P-Element transformation with period locus DNA restores rhythmicity to mutant arrhythmic Drosophila melanogaster. Cell (1984) 39:369-376.
- Siwicki KK, Eastman C, Petersen G, Rosbash M and Hall JC. Antibodies to the gene product of Drosphila reveal diverse tissue distribution and rhythmic changes in the visual system. Neuron (1988) 1:141-150.
- Hardin PE, Hall JC and Rosbash M. Circadian oscillations in period gene mRNA levels are transcriptionally regulated. Proc Natl Acad Sci USA (1992) 89:11711-11715.
- Seghgal A, Price JL, Man B and Young MW. Loss of circadian behavioral rhythms and per RNA oscillations in the Drosophila mutant timeless. Science (1994) 263(5153):1603-1606.
- Vosshall LB, Price JL, Sehgal A, Saez L and Young MW. Block in nuclear localization of period protein by a second clock mutation, timeless. Science (1994) 263(5153):1606-1609.
- Price JL, Blau J, Rothenfluh A, Abodeely M, Kloss B and Young MW. Double-time is a novel Drosophila clock gene that regulates PERIOD protein accumulation. Cell (1998) 94(1):83-95.
- Kojima S and Green CB. Circadian genomics reveal a role for post-transcriptional regulation in mammals. Biochemistry (2015) 54:124-133.
About the author
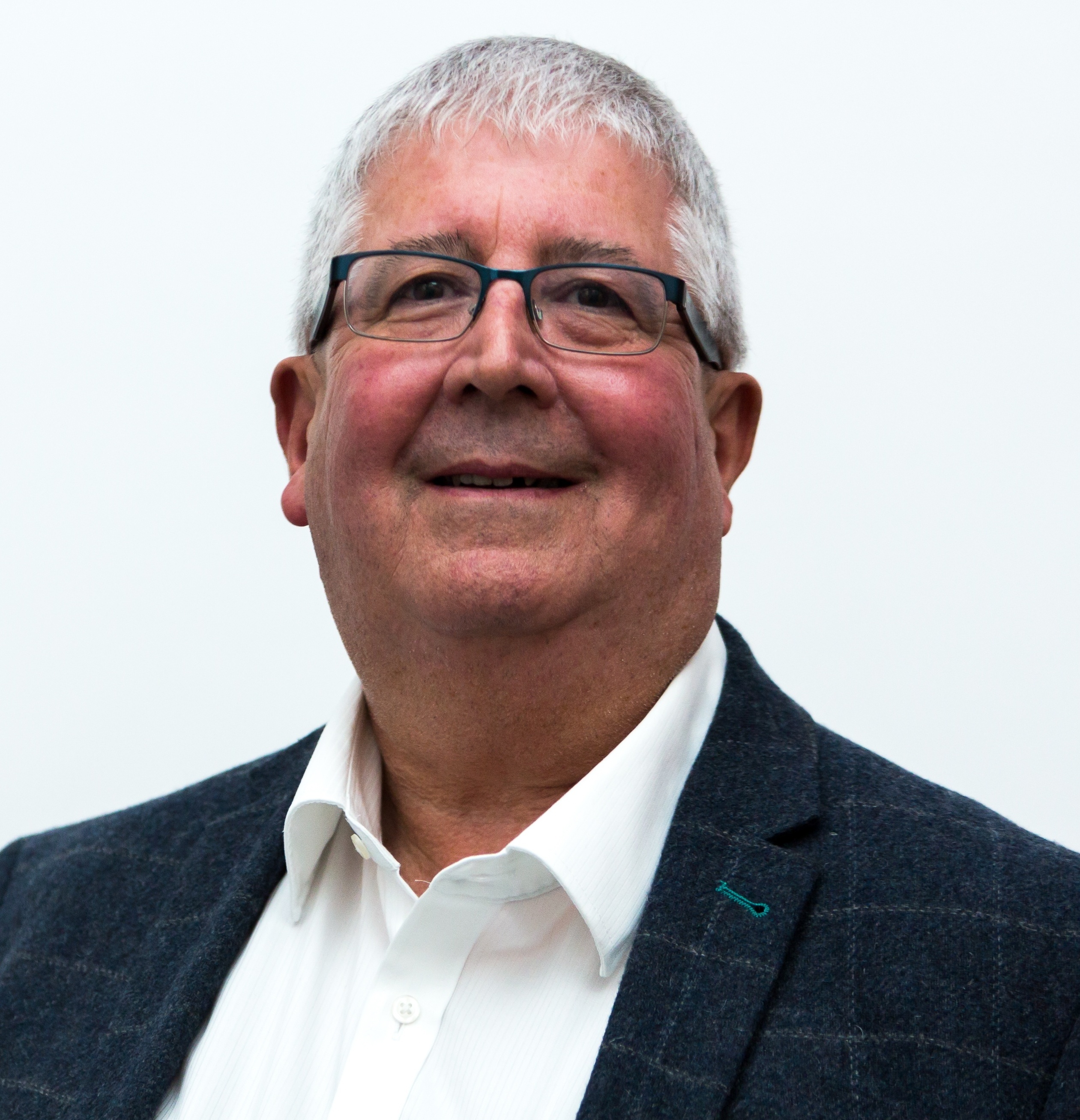
Kevin Moore
Kevin Moore is Head of Markets and Applications based out of Tecan’s head office in Männedorf, Switzerland. He heads the team tasked with bringing both products and application for the liquid handling to the market. Prior to joining Tecan in 2007, he was head of Compound Management and Technology project manager for the Neuroscience Research Centre of Merck & Co in the UK, where he worked for Merck for 20 years.