Keywords:
By Oliver Schmidt
As a nuclear protein present in most cell types, HMGB1 (high mobility group box 1) is a key mediator of the immune system in health and disease. Interest in HMGB1 has increased dramatically as the protein has been shown to be critical to the cell’s response to stress and plays a major role in many disease states, including infectious diseases, ischemia, immune disorders, neurodegenerative diseases, metabolic disorders, and, not least, cancer. Highly conserved in mammals, HMGB1 (also known as HMG-1 and amphoterin) is primarily located in the chromatin where it stabilizes chromosome structure and plays a key role in controlling gene expression.
During cell stress, HMGB1 can also be modified and translocated to the cytosol and released actively through secretion by immune cells, or passively by injured cells as the cell’s immediate cry for help. Its involvement in chromatin stability, gene expression, intracellular signaling, immune regulation, inflammation, cell death, and many other processes make HMGB1 a highly promising clinical biomarker and also an attractive target for therapy in what is becoming a wide-open area of translational medicine.
HMGB1 discovery and characterization
In 1973, Graham Goodwin and Ernest Johns at the Institute of Cancer Research in London published their discovery of a group of proteins found in calf thymus chromatin that they called High-Mobility Group (HMG) after their rapid migration rate in gel electrophoresis. Over the next twenty years, individual members of the HMG group were identified and characterized. In 2001, the HMG group members were renamed to HMGA, HMGN, and HMGB sub-members, including HMGB1 (High mobility group box 1). Their sensitivity to post-translational modification, such as phosphorylation,acetylation and redox-modifications, which controls their cellular distribution and function, resulted in a further revision of the nomenclature in 2014.
An alarmin that is central to survival through the inflammation response
HMGB1 is key to the evolution and survival of animals. As Professor Marco Bianchi, Professor of Molecular Biology at San Raffaele University, in Milan, Italy explains,
“HMGB1 is highly conserved in animals, and especially mammals, and is at the heart of inflammation, which is one of the most important mechanisms animals have developed in response to their environment – whether it be infection, physical trauma, or the development of unwanted tissue such as cancer.”
HMGB1 is initially located in the nucleus, where it has many functions, including stabilizing and regulating nucleosome function through histone binding, influencing transcription rates and gene expression, and supporting DNA repair.
HMGB1 is an alarmin, an endogenous member of the group of proteins called DAMPs (Danger Associated Molecular Patterns) and is released actively by immunocompetent cells (such as macrophages), or passively by dead, dying or damaged cells. Its action depends on post-translational modifications and, to a certain extent, redox state.
Passive release of unmodified HMGB1 signals the presence of dead cells and tissue damage. HMGB1 can also be actively released once it has been acetylated and translocated from the nucleus to the cytosol, followed by secretion as the cell’s first cry for help.
As it leaves the cell, completely reduced HMGB1 binds to a small chemokine called CXCL12, creating a complex that recruits immune cells. These cells produce oxygen reactive species to oxidize HMGB1, forming a disulfide bond and this isoform binds to a receptor, TLR4, which is a receptor for lipopolysaccharide, a component of bacteria. Professor Marco Bianchi continues,
“It is very interesting that the recruitment and activation of inflammatory cells are performed by the same protein but in different redox states. And it is also very interesting that cells react essentially in the same way to cell damage and attack by bacteria, leading to inflammation. Inflammation then initiates different programs to stop the damage and reconstruct the tissue.”
Professor Bianchi adds,
“A third redox form of HMGB1 involves oxidation of the cysteines to cysteine-sulfones, and acts as a marker of the resolution of the inflammation.”
HMGB1 is thus involved in many extracellular processes, including inflammation, immunity, cell migration, invasion, proliferation and differentiation, antimicrobial defense, and tissue regeneration. The protein may also be presented at the cell membrane to promote neurite outgrowth, platelet activation, or cell adhesion.
The complexity of cell death
HMGB1 can be used as a marker for DNA fragmentation related to apoptosis in macrophages, cancer cells and other cells, but the role of HMGB1 in apoptosis is complex. HMGB1 has been invoked in central apoptosis pathways and cancer cells can release HMGB1 during apoptosis. On the other hand, extracellular oxidized HMGB1 can induce apoptosis in cancer cells. Intracellular HMGB1 can also prevent apoptosis in response to a number of stimuli.
An invaluable biomarker and promising target for a multitude of diseases
HMGB1 has been implicated in a multitude of diseases and conditions, but we will look at just a few: sepsis, cancer, stroke, and arthritis.
Sepsis is a serious, life-threatening systemic inflammatory response syndrome often caused by microbial infection or an inflammatory trauma that leads to the release of pro-inflammatory cytokines, including HMGB1. Early response cytokines peak and return to baseline after 3–4h, which only provides a small window for diagnostics. HMGB1, on the other hand, is one of the delayed response cytokines and can be detected from 8h, and reaches plateau levels from 16 to 32h, making it much more valuable as a diagnostic marker.
Both a tumor suppressor and oncogene
Researchers have pinpointed HMGB1 as playing a major role in many cancers, including breast, colon, lung, prostate, skin, cervical, kidney, stomach, liver, pancreatic, bone, and blood cancer.
Depending on context, location and level of modification, HMGB1 acts as both a tumor suppressor and an oncogenic factor in tumorigenesis and cancer therapy. Nuclear HMGB1 can play a key role in tumor suppression, for example, by binding to tumor suppressors and also as an important architectural component in chromatin. Loss of HMGB1 results in genome instability and telomere shortening, which drives tumorigenesis. HMGB1 also stimulates autophagy, which is important in avoiding the genome instability and inflammation that promote tumorigenesis.
HMGB1 can be released from the cell, for example after hypoxia or other stimuli, and is involved in communication between tumor cells via several receptors, such as RAGE and TLR4. This communication boosts tumor growth by, for example, maintaining inflammation and promoting invasion, metastasis and angiogenesis. Inhibiting HMGB1 release and action can therefore block tumor growth and development.
Contributing both sensitivity and resistance to anticancer therapy
Depending on the type of anticancer therapy, cancer cell death can be immunogenic or non-immunogenic. Many factors determine the role of HMGB1 in the anticancer immune response, including receptor, death type, and redox state. For example, HMGB1 release contributes to anticancer immunity, but release during cell death may lead to immunogenic tolerance. Release during chemotherapy enhances the ability of remnant cancer cells to regrow and metastasize in a RAGE-dependent way. Inhibition of the HMGB1-RAGE pathway improves the effectiveness of chemotherapy.
On the other hand, intracellular HMGB1 can lead to resistance to anticancer therapy. Several chemotherapy agents increase HMGB1 expression, inhibiting apoptosis and autophagy, which determine cell fate in anticancer therapy. This makes HMGB1 a novel therapeutic target for chemotherapy resistance.
Case study: The promise of HMGB1 neutralization therapy in stroke
An EU-financed project, CounterStroke (www.counterstroke.se), is aiming to treat stroke with a novel approach that involves neutralizing HMGB1 early in the inflammation process by using Affibody molecules (http://www.affibody.se; engineered proteins significantly smaller than antibodies).
During stroke, tissue death releases HMGB1 and a ring of inflammation, called the penumbra, forms around the dead tissue and inflammatory cells infiltrate into the CNS. This is followed by active release of HMGB1, which enhances the inflammatory process and makes HMGB1 an excellent target for combating stroke. Professor Helena Erlandsson Harris at Karolinska Institute, in Stockholm, Sweden, who heads the project, explains, “The more inflammation the worse the outcome, and there are reports that total HMGB1 levels can correlate with the size of the lesion and the clinical outcome. Our project aims to define an Affibody molecule with the best neutralizing effect on HMGB1, ready to be taken to the clinical phase.”
The treatment window for the Affibody molecule could be trimmed in an elegant way, by designing an Affibody molecule with a short half-life, or tagging it to bind to albumin to extend its half-life. Treatment with a neutralizing Affibody molecule would have several advantages over current treatments that involve either physically removing the blood clot or pharmacological thrombolysis, which must be performed within four hours of the stroke and only when it is not caused by hemorrhaging. In contrast, the Affibody molecule could be used to treat stroke irrespective of the cause. “You could actually have a pre-packed syringe in the ambulance and start treatment as soon as you suspect a stroke. So it could be a complementary treatment to thrombolysis,” concludes Professor Erlandsson Harris.
Case study: Juvenile arthritis and the need for measuring isoforms
Professor Erlandsson Harris and her group at Karolinska Institute also have a strong interest in studying the role of actively released HMGB1 in juvenile idiopathic arthritis. At a frequency of 15 cases per 100 000 and year, this is the most common chronic inflammatory condition in children. Early work indicated that high levels of total HMGB1 correlated with a young age of disease onset. In their latest work, they have found that HMGB1 is actively released in chronically inflamed joints and that the release involves neutrophils and is mainly dependent on acetylation. This has highlighted the need to look HMGB1 at a higher resolution that examines the isoforms resulting from post-translational modifications. Professor Erlandsson Harris adds, “Our group is now aiming to get support for HMGB1 as a prognostic or diagnostic marker by increasing the cohort size so that we can correlate results with clinical markers.”
The challenge of measuring HMGB1
These and a multitude of other insights into the complex role that HMGB1 plays in disease has driven the analysis of HMGB1 from tedious Western Blotting, through time saving, high throughput ELISA to advanced Liquid Chromatography/Mass Spectrometry that can measure isoforms. We will look at these methods in the second article in this series.
Further reading
Andersson U, Tracey KJ. HMGB1 is a therapeutic target for sterile inflammation and infection. Annu Rev Immunol. 2011;29:139-62. [PMID: 21219181]
Goodwin GH, Johns EW. Isolation and characterisation of two calf-thymus chromatin non-histone proteins with high contents of acidic and basic amino acids. Eur J Biochem. 1973 Dec 3;40(1):215–219. [PMID: 4772679]
Kang R. et al. HMGB1 in health and disease. Mol Aspects Med. 2014 Dec;40:1-116. [PMID: 25010388]
Lundbäck P et al. Characterization of the Inflammatory Properties of Actively Released HMGB1 in Juvenile Idiopathic Arthritis. Antioxid Redox Signal. 2016 Apr 20;24(12):605-19. [PMID: 25532033]
Venereau E et al. HMGB1 and leukocyte migration during trauma and sterile inflammation. Mol Immunol. 2013 Aug;55(1):76-82. [PMID: 23207101]
Yu Y. et al. Oxidative stress-mediated HMGB1 biology. Front Physiol. 2015 Apr 7;6:93 [PMID: 25904867]
About the author
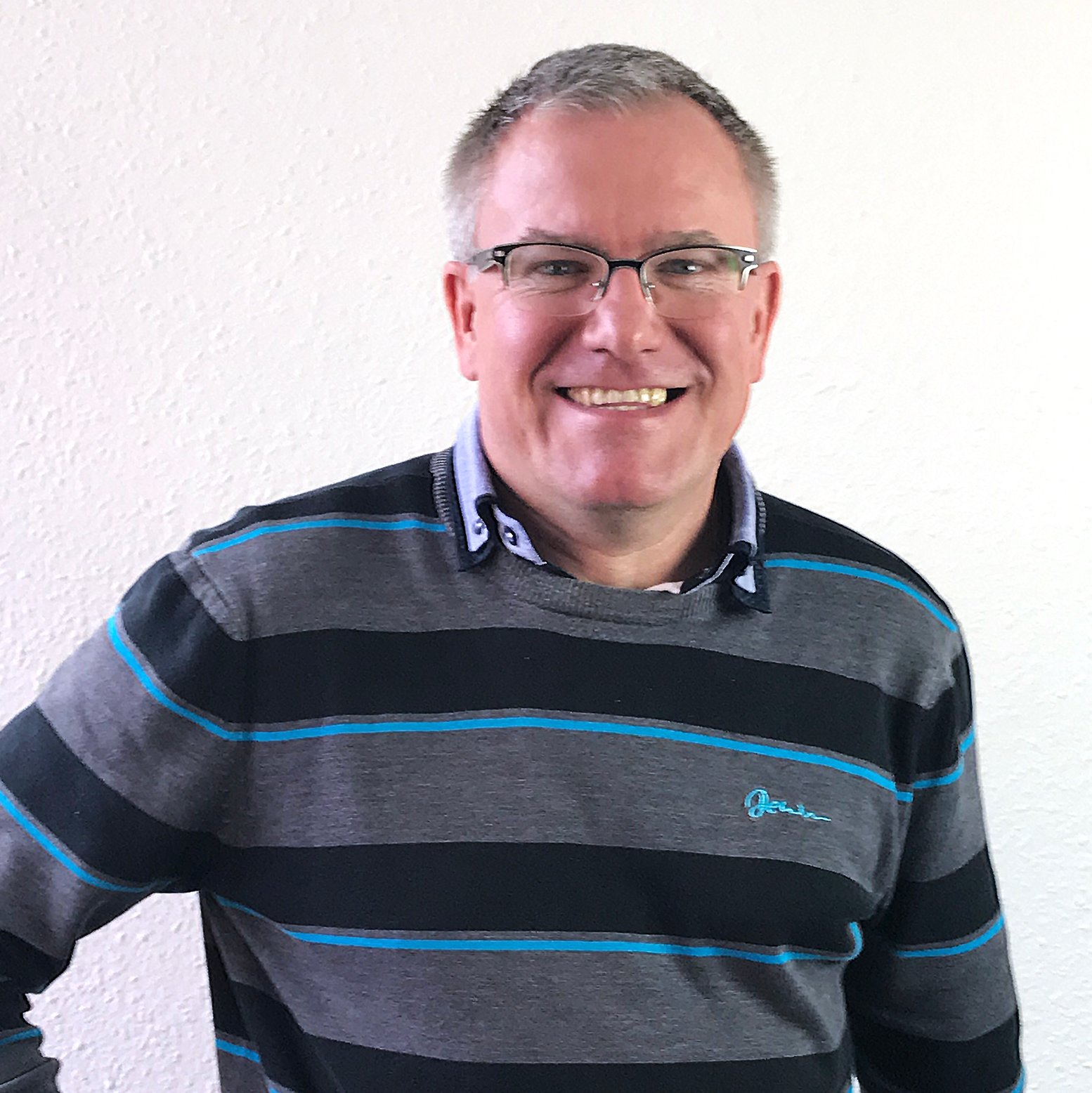
Oliver Schmidt
Oliver Schmidt is product manager for research reagents, neurodegeneration, and bone and mineral products at TECAN-IBL in Hamburg with more than 10 years’ experience in bringing these products to the Life Science and academic community. He studied Environmental Engineering at the Technical University of Hamburg-Harburg and joined TECAN-IBL in 2005.